ELECTROREDUCTION OF NITROUS OXIDE WITH NANOSTRUCTURED SNO2 MATERIALS
NANOSCIENCE
Lab: LCC
Duration: From 6 to 8 months (Mob Internship)
Latest starting date: 02/01/2025
Localisation: Molecules and Composites for Optics (LCC) - Nanostructures and Organometallic Chemistry (LPCNO)
Laboratoire de Chimie de Coordination du CNRS (LCC-CNRS)
205, route de Narbonne
31400 TOULOUSE - FRANCE
Supervisors:
Nicolas QUERYIAUX nicolas.queyriaux@lcc-toulouse.fr
Nuria ROMERO nuria.romero@lcc-toulouse.fr
Work package:
Nitrous oxide is an important atmospheric pollutant emitted by human activities, acting both as the third most
important greenhouse gas and the main ozone-depleting agent. Its tropospheric concentration is constantly
rising when compared to pre-industrial levels, driven by anthropogenic perturbations of the nitrogen cycle: large-
scale use of synthetic fertilizers in modern agriculture, chemical-processing manufactures, fossil fuels burning...[1]
Thermal decomposition, direct catalytic decomposition and selective catalytic reduction of N2O have emerged
as interesting strategies to mitigate N2O emissions and decrease its environmental footprints.[2] However, they
typically suffer from energy-intensive processes and are characterized by a high level of waste production. An
alternative option lies in the utilization of electrocatalytic N2O decomposition, which reduces nitrous oxide at an
electrode surface. Such approach displays significant advantages, including room-temperature reaction, low
energy consumption and absence of external reducing agents.
Beyond the typical noble metal-based electrodes (Pt, Pd),[3] alternative electroactive materials relying on
abundant metal oxides have been explored. Bulk Tin(IV) oxide (SnO2), in particular, have shown promising
catalytic properties when employed in aqueous media: faradaic yields of ca. 75% for N2O reduction and high
selectivity against proton reduction.[4] Nanostructuration of SnO2 is therefore a promising pathway to obtain
highly active and selective electrocatalysts. Small and well dispersed core-shell Sn@SnO2 nanoparticles can be
obtained according to Scheme 1
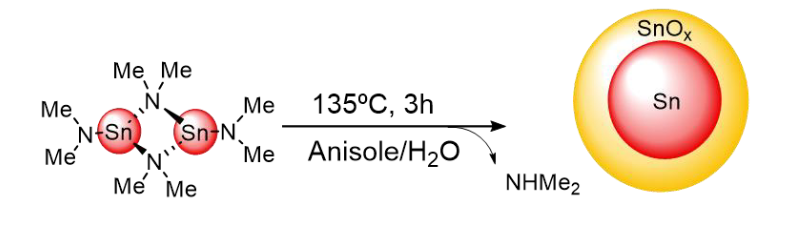
References:
.[1] V. Masson-Delmotte, P. Zhai, A. Pirani, S. L. Connors, C. Péan, S. Berger, N. Caud, Y. Chen, L. Goldfarb, M. I. Gomis, M. Huang, K.
Leitzell, E. Lonnoy, J. B. R. Matthews, T. K. Maycock, T. Waterfield, Ö. Yelekçi, R. Yu, B. Zhou, Eds. , Climate Change 2021: The Physical
Science Basis. Contribution of Working Group I to the Sixth Assessment Report of the Intergovernmental Panel on Climate Change,
Cambridge University Press, Cambridge, United Kingdom and New York, NY, USA, 2021.
[2] X. Wu, J. Du, Y. Gao, H. Wang, C. Zhang, R. Zhang, H. He, G. (Max) Lu, Z. Wu, Chem. Soc. Rev. 2024, 53, 8379–8423.
[3] A. Kudo, A. Mine, Journal of Electroanalytical Chemistry 1996, 408, 267–269.
[4] A. Kudo, A. Mine, Applied Surface Science 1997, 121–122, 538–542.
[5] C. Nayral, E. Viala, P. Fau, F. Senocq, J.-C. Jumas, A. Maisonnat, B. Chaudret, Chemistry – A European Journal 2000, 6, 4082–4090.
Areas of expertise:
In this internship, investigation of such materials towards N2O electroreduction will be conducted. Effects of
doping, size and surface tuning, support nature (carbonaceous vs conducting glass) and experimental conditions
(pH, substrate feed...) will systematically be examined to gain insight into the main parameters driving catalysis
in this family of materials.
Required skills for the internship:
Solid skills in nanoparticles, synthesis and material characterization (TEM/HRTEM, IR, XRD, XPS, ICP, NMR, etc.), electrode assembly and
electrocatalysis will be acquired.